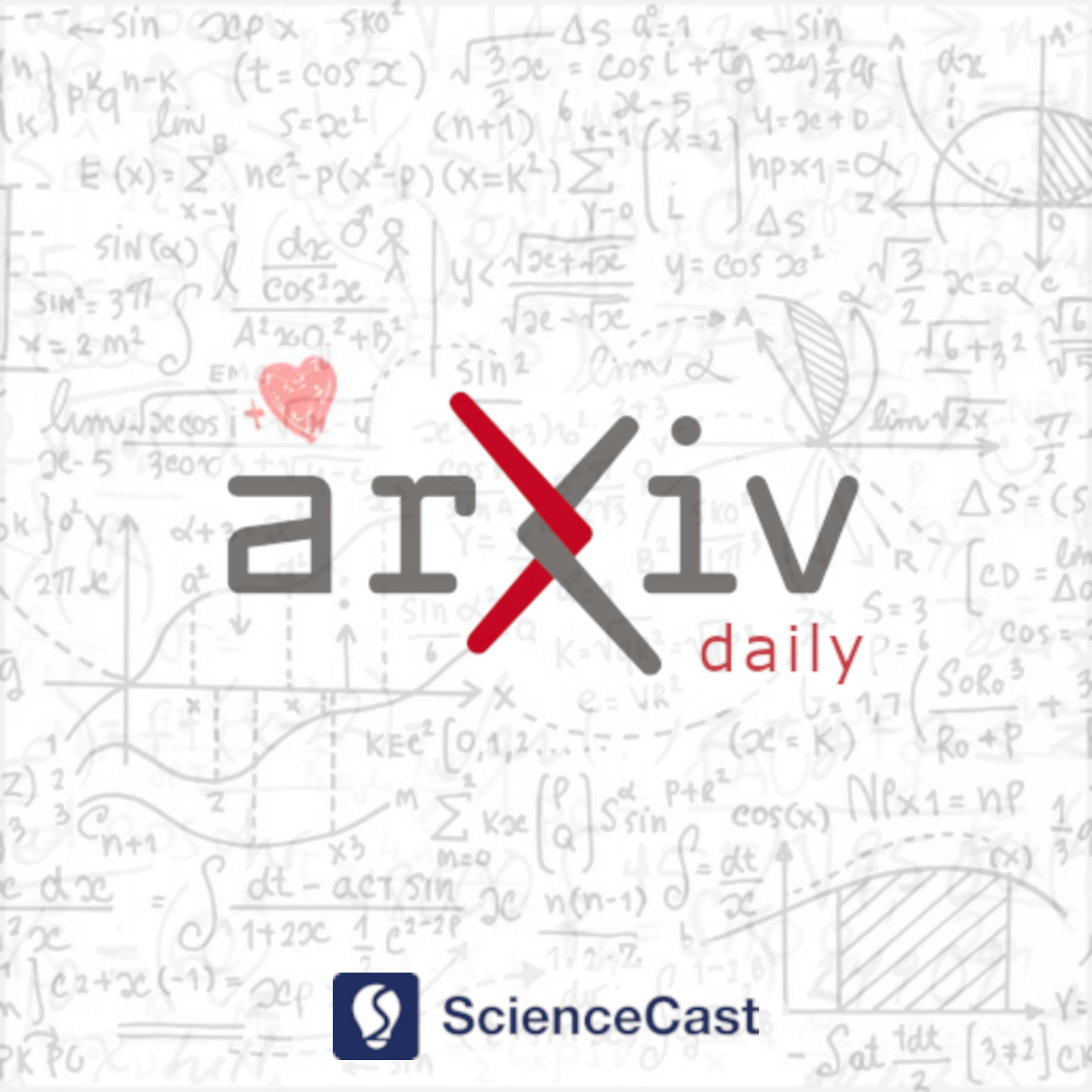
Combinatorics (math.CO)
Wed, 12 Jul 2023
1.Hochschild polytopes
Authors:Vincent Pilaud, Daria Poliakova
Abstract: The $(m,n)$-multiplihedron is a polytope whose faces correspond to $m$-painted $n$-trees, and whose oriented skeleton is the Hasse diagram of the rotation lattice on binary $m$-painted $n$-trees. Deleting certain inequalities from the facet description of the $(m,n)$-multiplihedron, we construct the $(m,n)$-Hochschild polytope whose faces correspond to $m$-lighted $n$-shades, and whose oriented skeleton is the Hasse diagram of the rotation lattice on unary $m$-lighted $n$-shades. Moreover, there is a natural shadow map from $m$-painted $n$-trees to $m$-lighted $n$-shades, which turns out to define a meet semilattice morphism of rotation lattices. In particular, when $m=1$, our Hochschild polytope is a deformed permutahedron whose oriented skeleton is the Hasse diagram of the Hochschild lattice.
2.Projective dimension of weakly chordal graphic arrangements
Authors:Takuro Abe, Lukas Kühne, Paul Mücksch, Leonie Mühlherr
Abstract: A graphic arrangement is a subarrangement of the braid arrangement whose set of hyperplanes is determined by an undirected graph. A classical result due to Stanley, Edelman and Reiner states that a graphic arrangement is free if and only if the corresponding graph is chordal, i.e., the graph has no chordless cycle with four or more vertices. In this article we extend this result by proving that the module of logarithmic derivations of a graphic arrangement has projective dimension at most one if and only if the corresponding graph is weakly chordal, i.e., the graph and its complement have no chordless cycle with five or more vertices.
3.Asymmetry of 2-step Transit Probabilities in 2-Coloured Regular Graphs
Authors:Ron Gray, J. Robert Johnson
Abstract: Suppose that the vertices of a regular graph are coloured red and blue with an equal number of each (we call this a balanced colouring). Since the graph is undirected, the number of edges from a red vertex to a blue vertex is clearly the same as the number of edges from a blue vertex to a red vertex. However, if instead of edges we count walks of length $2$, then this symmetry disappears. Our aim in this paper is to investigate how extreme this asymmetry can be. Our main question is: Given a $d$-regular graph, for which pairs $(x,y)\in[0,1]^2$ is there a balanced colouring for which the probability that a random walk starting from a red vertex stays within the red class for at least $2$ steps is $x$, and the corresponding probability for blue is $y$? Our most general result is that for any $d$-regular graph, these pairs lie within the convex hull of the $2d$ points $\left\{\left(\frac{l}{d},\frac{l^2}{d^2}\right),\left(\frac{l^2}{d^2},\frac{l}{d}\right) :0\leq l\leq d\right\}$. Our main focus is the torus for which we prove both sharper bounds and existence results via constructions. In particular, for the $2$-dimensional torus, we show that asymptotically, the region in which these pairs of probabilities can lie is exactly the convex hull of: \[ \left\{\left(0,0\right),\left(\frac{1}{2},\frac{1}{4}\right),\left(\frac{3}{4},\frac{9}{16}\right),\left(\frac{1}{4},\frac{1}{2}\right),\left(\frac{9}{16},\frac{3}{4}\right),\left(1,1\right)\right\} \]
4.Relative Fractional Independence Number and Its Applications
Authors:Sharareh Alipour, Amin Gohari
Abstract: We define the relative fractional independence number of two graphs, $G$ and $H$, as $$\alpha^*(G|H)=\max_{W}\frac{\alpha(G\boxtimes W)}{\alpha(H\boxtimes W)},$$ where the maximum is taken over all graphs $W$, $G\boxtimes W$ is the strong product of $G$ and $W$, and $\alpha$ denotes the independence number. We give a non-trivial linear program to compute $\alpha^*(G|H)$ and discuss some of its properties. We show that $$\alpha^*(G|H)\geq \frac{X(G)}{X(H)},$$ where $X(G)$ can be the independence number, the zero-error Shannon capacity, the fractional independence number, the Lov'{a}sz number, or the Schrijver's or Szegedy's variants of the Lov'{a}sz number of a graph $G$. This inequality is the first explicit non-trivial upper bound on the ratio of the invariants of two arbitrary graphs, as mentioned earlier, which can also be used to obtain upper or lower bounds for these invariants. As explicit applications, we present new upper bounds for the ratio of the zero-error Shannon capacity of two Cayley graphs and compute the Haemers number of certain Johnson graphs. Moreover, we show that the relative fractional independence number can be used to present a stronger version of the well-known No-Homomorphism Lemma. The No-Homomorphism Lemma is widely used to show the non-existence of a homomorphism between two graphs and is also used to give an upper bound on the independence number of a graph. Our extension of the No-Homomorphism Lemma is computationally more accessible than its original version.
5.Smoothed Analysis of the Komlós Conjecture: Rademacher Noise
Authors:Elad Aigner-Horev, Dan Hefetz, Michael Trushkin
Abstract: The {\em discrepancy} of a matrix $M \in \mathbb{R}^{d \times n}$ is given by $\mathrm{DISC}(M) := \min_{\boldsymbol{x} \in \{-1,1\}^n} \|M\boldsymbol{x}\|_\infty$. An outstanding conjecture, attributed to Koml\'os, stipulates that $\mathrm{DISC}(M) = O(1)$, whenever $M$ is a Koml\'os matrix, that is, whenever every column of $M$ lies within the unit sphere. Our main result asserts that $\mathrm{DISC}(M + R/\sqrt{d}) \leq 1 + O(d^{-1/2})$ holds asymptotically almost surely, whenever $M \in \mathbb{R}^{d \times n}$ is Koml\'os, $R \in \mathbb{R}^{d \times n}$ is a Rademacher random matrix, $d = \omega(1)$, and $n = \tilde \omega(d^{5/4})$. We conjecture that $n = \omega(d \log d)$ suffices for the same assertion to hold. The factor $d^{-1/2}$ normalising $R$ is essentially best possible.